Novel, fascinating aspects of quantum effects in two-dimensional materials are reported in two new publications by Prof. Dante Kennes and coworkers in Germany and the United States.
Quantum matter is one of the main fascinations of Prof. Dante Kennes – professor for Theoretical Condensed Matter Physics at RWTH Aachen University – and the reason why he is so excited about two-dimensional materials. “Two-dimensional materials are a perfect playground for studying exotic quantum effects caused by the interaction between particles”, says Kennes, “for one, because there are so many different ways in which we can tune their properties. For example, stacking different atomically thin layers on top of each other can dramatically alter their properties, as well as playing with the twist angle between the layers.”
The idea of engineering the properties of a stack of 2D materials by acting on the twist angle is now known as twistronics – a field that has been literarily booming in the past years, after the discovery that twisted bilayer graphene can become superconducting due to the interaction between the layers. The challenge for scientist is to find out precisely what happens when two atomically thin layers are stacked and twisted, which changes can be induced and how they can be tuned. Twistronics is a field that often requires the close collaboration between theorists and experimentalists, as in the case of the two recent papers published by Kennes and colleagues from Germany and the United States [1,2].
Moiré metrology
The first of these two publications introduces the concept of ‘moiré metrology’, a groundbreaking method to map the interaction between layers by studying the spatial patterns that emerge when two layers of a material are stacked and twisted – the so-called moiré superlattice. The notion at the heart of moiré metrology is that when two atomic layers are placed on top of each other they do not remain still, but stretch and compress in search of stable configurations determined by the interaction between the layers. The work by Kennes & coworkers shows how information about this interaction can be extracted from the fine spatial patterns that emerge after atomic relaxation [1].
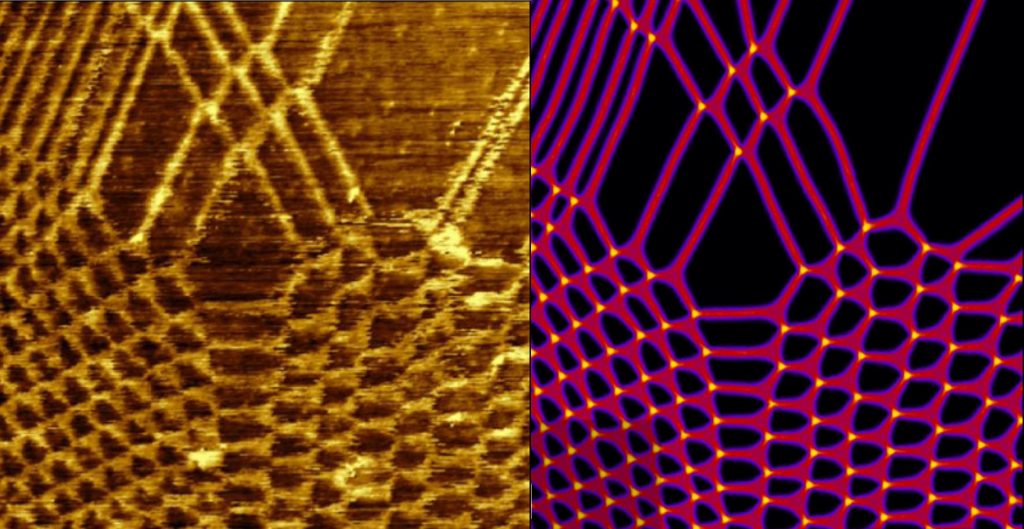
A programmable topological quantum material
The second paper demonstrates that four-layer rhombohedral graphene is a new material for realizing flat-band-based quantum phenomena and topological states. Rhombohedral graphene is a material known since many years, which can be formed by simply sliding with respect to each other the individual layers of bernal graphene, the more stable and common form of graphite. The problem is that in this way rhombohedral graphene is unstable and can easily transform back to bernal graphene. The new work demonstrates that a small twist between the two layers of bilayer graphene can result in large (micrometer-scale) regions of uniform rhombohedral four-layer graphene. Furthermore, the work shows that the electrons confined in the rhombohedral graphene regions show flat-energy bands – the right conditions to observe quantum phenomena – and, most interestingly, show topological properties in the presence of an external electric field [2]. This makes the new material a programmable topological quantum material, with possible ramifications for topological quantum computing.
“It is fascinating to see how competing structural and electronic interactions can affect the macroscopic properties of a few graphene layers,” says Dante Kennes. “We are currently investigating whether similar phenomena can be observed in other two-dimensional heterostructures and whether the concept can be extended to thicker samples consistent of many layers in which twisting introduced novel topological three-dimensional phases.”
Prof. Dante Kennes is also visiting scientist at the Max Planck Institute for the Structure and Dynamics of Matter in Hamburg (MPSD). More information about the two papers can be found in the official press releases by MPSD.
References:
- Moiré metrology of energy landscapes in van der Waals heterostructures, D. Halbertal, N. R. Finney, S. S. Sunku, A. Kerelsky, C. Rubio-Verdú, S. Shabani, L. D. Xian, S. Carr, S. Chen, C. Zhang, L. Wang, D. Gonzalez-Acevedo, A. S. McLeod, D. Rhodes, K. Watanabe, T. Taniguchi, E. Kaxiras, C. R. Dean, J. C. Hone, A. N. Pasupathy, D. M. Kennes, A. Rubio, D. N. Basov, Nature Communications 12, 242 (2021). [Press release by MPSD]
- Moiréless correlations in ABCA graphene
A. Kerelsky, C. Rubio-Verdú, L. D. Xian, D. M. Kennes, D. Halbertal, N. Finney, L. Song, S. Turkel, L. Wang, K. Watanabe, T. Taniguchi, J. Hone, C. Dean, D. Basov, A. Rubio, A. N. Pasupathy, Proceedings of the National Academy of Sciences of the United States of America 118 (4), e2017366118 (2021). [Press release by MPSD]